
Protein MICAL1 Plays a Key Role in Cellular Dynamics by Controlling the Cytoskeleton
Researchers from the Faculty of Science at Charles University, three institutes of the Czech Academy of Sciences (IMG, IBT and IOCB), BIOCEV and CEITEC have made a remarkable advancement in understanding how cells regulate their inner framework. In a study recently published in Nature Communications, the team has uncovered the precise molecular mechanisms behind the protein MICAL1, which plays a crucial role in maintaining cell shape and movement. Their findings offer a detailed insight into MICAL1’s function in controlling actin filaments—the cell's flexible “skeleton”—and open up new possibilities for developing treatments to address diseases linked to MICAL dysfunction.
Every cell in our body has a framework called the cytoskeleton—a dynamic network of protein filaments that provides structure, enables movement, and helps the cell respond to its environment. One of the most important components of this cytoskeleton is actin filaments. These long, thin strands of protein are like the cables and girders of a construction site, providing cells with both structure and flexibility. Actin filaments are vital for processes like muscle contraction, nerve cell connectivity, and cell division. However, to perform these roles effectively, the cytoskeleton must constantly reshape itself in response to changing cellular needs.
A family of proteins called MICAL proteins plays a critical role in reshaping actin filaments. Acting as cellular “scissors,” MICAL proteins selectively break down actin filaments, enabling cells to change shape and move as needed. However, it is essential that MICAL proteins remain inactive when they are not required, as uncontrolled activity would disrupt the cell’s structural integrity. This new study reveals how MICAL1 remains “locked” in an inactive state until a cellular signal prompts it to engage, preventing accidental damage to the cell’s internal structure. When activated, MICAL1 binds to actin filaments and breaks them down through a process called depolymerization, allowing the cell to adjust its shape and respond dynamically to its environment.
(b) Detailed view of the MICAL1 protein structure in its autoinhibited state.

"We used cutting-edge cryo-electron microscopy to capture MICAL1 at the atomic level and show that its function is tightly controlled by specific molecular interactions, ensuring it only activates when needed," explains Matej Horvath, the study’s first author and now a postdoctoral research fellow at the Okinawa Institute of Science and Technology. "Without this regulation, MICAL1’s activity could harm the cell, highlighting the critical need for precise control in cellular processes."
“This discovery brings new hope for understanding diseases where cellular structure breaks down,” says Daniel Rozbesky, head of the Laboratory of Structural Neurobiology at the BIOCEV Research Centre. “Our atomic-level cryoEM structure suggests that in certain patients with a specific form of epilepsy, pathogenic variants of MICAL1 become hyperactive due to a failure in maintaining their inactive state. Moving forward, we plan to explore ways to restore proper MICAL1 regulation, with the potential to provide relief for patients affected by this condition.”
Publication: https://www.nature.com/articles/s41467-024-54131-2
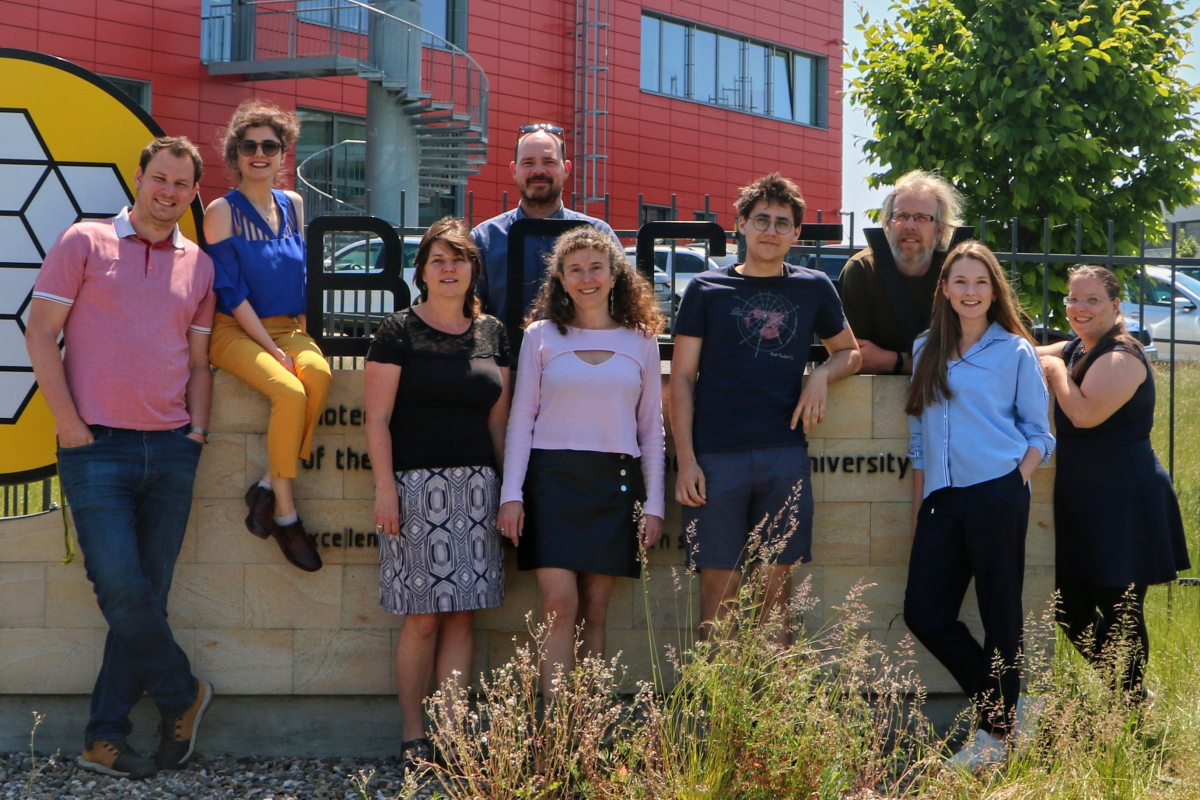